Microbes have a variety of mechanisms for resisting antimicrobials. Some mechanisms are intrinsic (naturally produced by the organism), some are induced by certain conditions or drugs, some are genetically acquired mutations, and others can be passed from organism to organism. The last two mechanisms are one reason why preventing the formation of resistant organisms is important. Once an organism has a genetic mutation that allows it to survive our first-line antibiotics, it will produce more organisms with that same mutation. Similarly, if an organism can pass a mutation to other organisms, every available organism will soon have it.
Some organisms, like Pseudomonas, can have multiple resistance mechanisms, making them harder to treat.
The most commonly clinically found resistance mechanisms are listed below.
- Enzymatic degradation – when enzymes hydrolyze or render antibiotic molecules ineffective (e.g., beta-lactamases)
- Change in the binding site – when the target at which an antibiotic binds and exerts it’s antibacterial mechanism changes (e.g., with the MecA gene in Staphylococci)
- Loss of porin channels – reduces the antibiotic’s capacity to enter the bacterial cell
- Increased efflux pumps – reduce the antibiotic’s capacity to remain in the bacterial cell
Visual Chart of Beta-Lactamases
Gram-positive Resistance Determinants / Mechanisms
Mutation of 23S rRNA has been established as a linezolid resistance mechanism. Although ribosomal proteins L3 and L4 are located further away from the bound drug, mutations in specific regions of these proteins are increasingly associated with linezolid resistance.
Vancomycin resistance is caused by an altered peptidoglycan terminus (d-ala-d-lac instead of the usual d-ala-d-ala) resulting in reduced vancomycin binding and failure to prevent cell wall synthesis.
Gram-negative Resistance Determinants / Mechanisms
Genotype | Resistance mechanism | Resistance to | Alternative antibiotics | Common Microbes |
ampC | Penicillinase, cephalosporinase (class C serine beta-lactamase) Can be inducible, genetic mutations, or plasmid-mediated | Penicillins Most cephalosporins | Cefepime, Ceftolozane-tazobactam Ertapenem Imipenem-cilastatin Meropenem Aminoglycosides TMP-SMX FQs | “HECK Yes” – Hafnia alvei, Enterobacter cloacae, Citrobacter freundii, Klebsiella aerogenes, and Yersinia enterocolitica (previously SPACE/SPICE – See Kohlmann et al. Species-specific mutation rates for ampC derepression in Enterobacterales with chromosomally encoded inducible AmpC β-lactamase. for more on change) Inducible resistance in Enterobacter cloacae, Citrobacter freundii, non-inducible chromosomal resistance due to mutations in Escherichia coli, Shigella spp., Acinetobacter baumannii), and plasmid-mediated resistance in Klebsiella pneumoniae, Escherichia coli, Salmonella spp. |
CTX-M, SHV, TEM (ESBLs) | Penicillinase, cephalosporinase (class A serine beta-lactamase) | Penicillins Penicillin-BLI combinations Most cephalosporins (except cephamycins) Aztreonam | Ceftolozane-tazobactam Ertapenem Imipenem-cilastatin Meropenem Aminoglycosides | The HECK YES/SPACE/SPICE bugs can have ESBL too. Most commonly Escherichia coli, Klebsiella pneumoniae, Acinetobacter baumannii. Pseudomonas aeruginosa can also be ESBL. |
KPC | Penicillinase, cephalosporinase carbapenemase (class A serine beta-lactamase) | Penicillins Penicillin-BLI combinations Cephalosporins Carbapenems Aztreonam | Ceftazidime-avibactam Meropenem-vaborbactam Imipenem-cilastatin-relebactam Cefiderocol Certain Aminoglycosides | KPC was named for Klebsiella pneumoniae |
OXA-48 OXA-48-like | Penicillinase, carbapenemase (class D serine beta-lactamase) | Penicillins Penicillin-BLI combinations Carbapenems Aztreonam | Ceftazidime-avibactam Cefiderocol Aminoglycosides | SPACE/SPICE/Heck Yes – Same as above, but most commonly in Klebsiella pneumoniae. |
IMP, VIM, NDM | Penicillinase, cephalosporinase carbapenemase (class B metallo-beta-lactamase) | Penicillins Penicillin-BLI combinations Cephalosporins Carbapenems | Aztreonam + Ceftazidime-avibactam Cefiderocol | Same as above, but most commonly in Escherichia coli, K. pneumoniae, Enterobacter cloacae, |
AmpC or ESBL?
ESBL | AmpC | Both | |
ceftriaxone, cefotaxime, ceftazidime | I/R | I/R | I/R |
cefoxitin | S | I/R | I/R |
cefepime | S | S | I/R |
Inhibitors (pip/tazo, amp/sulbactam, amox/clav) | S | I/R | I/R |
Treatment | Carbapenem | Cefepime or Carbapenem | Carbapenem |
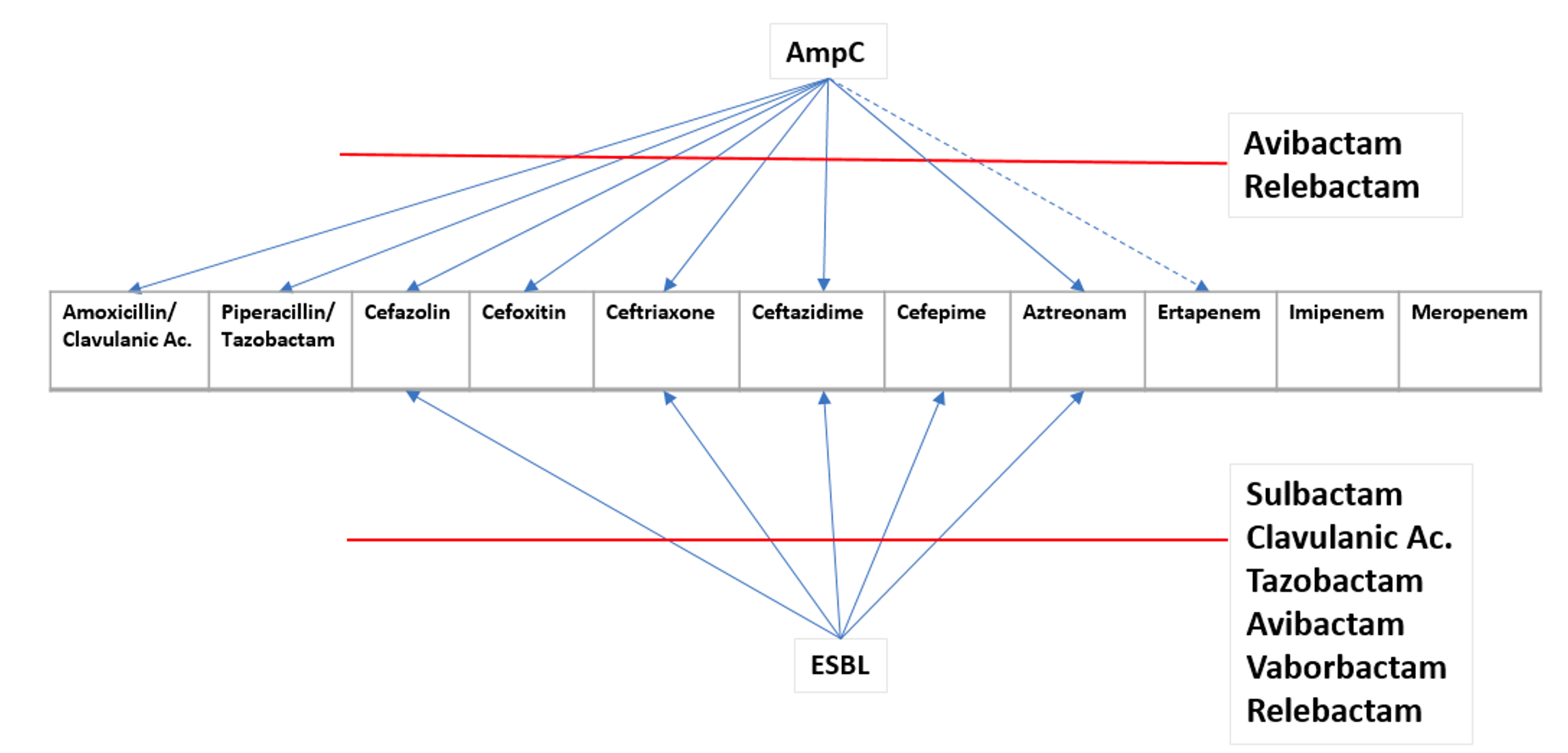
Why not Cefepime for ESBL?
- Majority of outcome data does not support empiric use of cefepime for ESBLs, especially for isolates with higher MICs that may be reported as susceptible (Patel HB, Lusk KA, Cota JM. The Role of Cefepime in the Treatment of Extended-Spectrum Beta-Lactamase Infections infections, (J Pharm Pract. 2019 Aug;32(4):458-463. doi: 10.1177/0897190017743134. Epub 2017 Nov 22. PMID: 29166830.)
- Outcome data that compared cefepime to carbapenems found higher mortality with cefepime use
- Cefepime should be avoided for non-urinary, severe ESBL infections due to isolates with MIC >1 treated with traditional cefepime dosing
- Cefepime may potentially be used as definitive therapy for treatment, IF isolate has low MIC ≤1 to cefepime and doses are optimized.
Why not Zosyn for ESBL?
- Zosyn may be useful for E. coli ESBLs in the urinary tract, but data does not support it’s usage otherwise.
- Tazobactam’s activity is reduced when there is a high concentration of bacteria (an inoculum effect), so it’s not as useful for systemic infections anyway.
- There are limited studies comparing Zosyn to carbapenems, and most found Zosyn inferior (a major one is the MERINO trial, Harris PNA, et al. JAMA 2018;320:984-94), however these studies haven’t always been the most targeted.
- If a patient is improving on Zosyn and they get an ESBL culture positive, they may not need to change antibiotics, but the jury is still out.
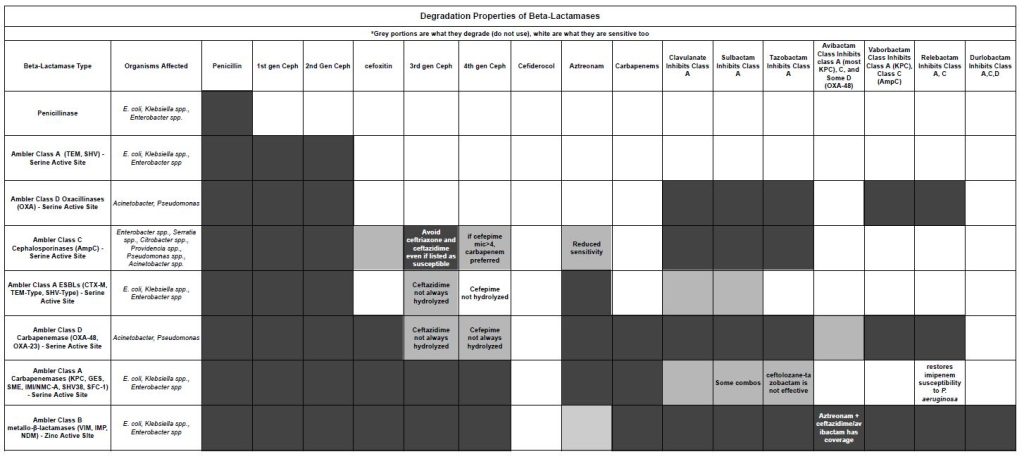
This chart may look better as a PDF.
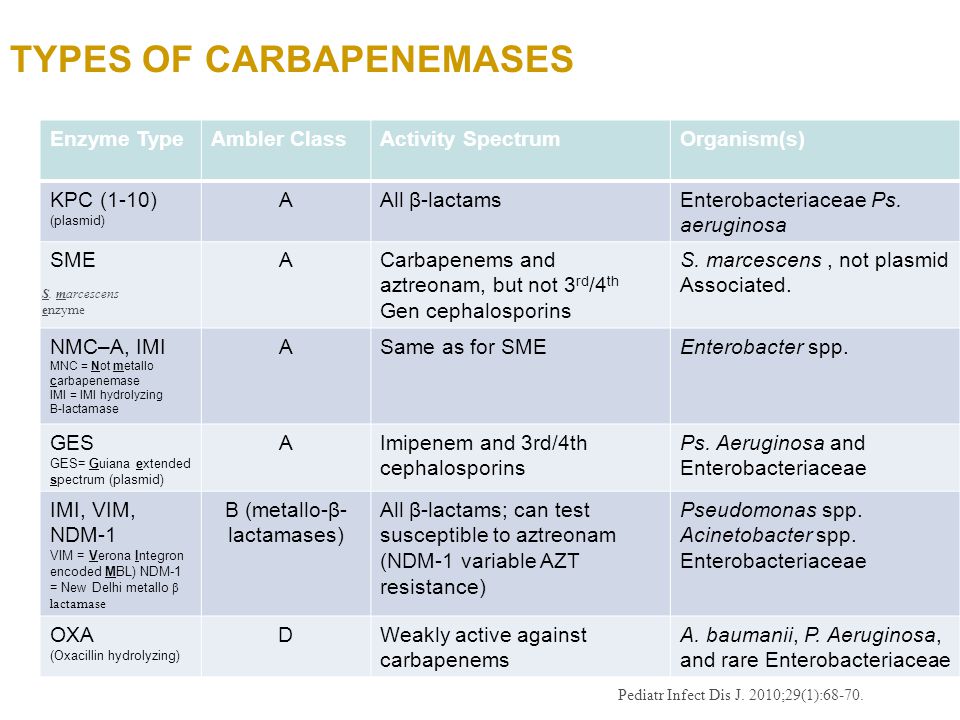
Inducible Clindamycin Resistance
Clindamycin has the most commonly referenced inducible resistance mechanism. In vitro routine tests for clindamycin susceptibility may fail to detect inducible clindamycin resistance due to erm genes resulting in treatment failure, thus necessitating the need to detect such resistance by a simple D test on routine basis. A positive D test (see Figure) indicates the presence of macrolide-inducible resistance to clindamycin produced by an inducible methylase that alters the common ribosomal binding site for macrolides, clindamycin and the group B streptogrammins (quinupristin) (Woods, 2009) .
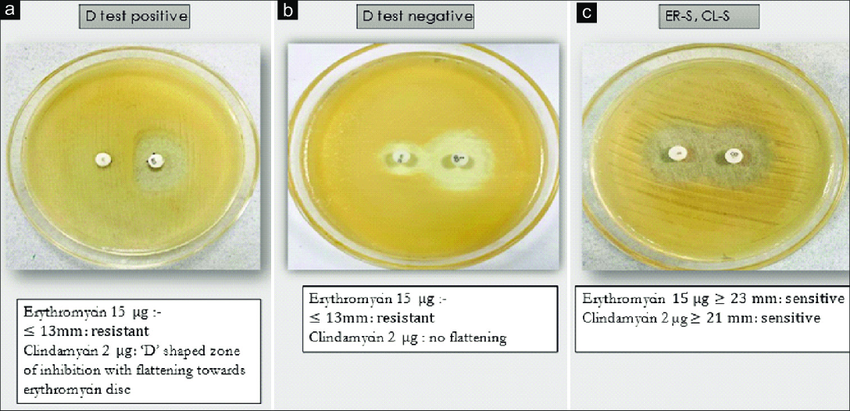
The inducible methylase system is regulated at the level of mRNA translation (translational attenuation) rather than gene transcription. The gene is transcribed to mRNA constitutively, but the mRNA cannot be read into protein without the presence of a translational inducer. When a macrolide molecule capable of inducing the methylase translation binds to its target site in a ribosome, the “inhibited.”
Resistance Mechanisms by Bug
*modified from US Pharmacist
Multidrug-Resistant Enterococcus: Enterococci, which are predominantly benign gut bacteria, may be the best-known family of microbes equipped with intrinsic and acquired antibiotic resistance. The two most clinically relative are E faecium and Enterococcus faecalis. These organisms can have multiple acquired resistance mechanisms, resulting in multidrug resistance. High levels of resistance may exist to beta-lactams (through beta-lactamase enzymes and altered penicillin-binding proteins), vancomycin (via changes in peptidoglycan synthesis via vanA/vanB; known as VRE), aminoglycosides (through enzymatic degradation), and linezolid from target rRNA alterations.
Ampicillin remains the drug of choice for susceptible Enterococcus infections, but few options remain to treat systemic multidrug-resistant Enterococcus infections; these include linezolid, daptomycin, and tigecycline.
Enterococcus faecalis is the most commonly found clinically, but E. faecium is the most resistance. Almost all VRE are E. faecium . I remember it because “fácil” is easy in Spanish and faecalis is more fácil to kill.
Methicillin-Resistant Staphylococcus aureus (MRSA) and mecA: MRSA first appeared in the early 1960s, shortly after the introduction of methicillin. As with most gram-positive organisms, the cell wall serves as the main target for antimicrobial agents and fosters acquired antimicrobial resistance. For S aureus to be considered MRSA, the bacterium must possess the mecA gene, which encodes for a structural change in penicillin-binding protein 2a (PBP2a). This change prevents beta-lactam antibiotics from binding to the cell wall.
Laboratory testing for S aureus susceptibility to methicillin is not commonly performed, owing to the instability of methicillin plates; rather, MRSA is determined by a similar beta-lactam: oxacillin. The Clinical Laboratory Standards Institute defines methicillin resistance as an oxacillin mean inhibitory concentration of at least 4 mcg/mL, and isolates resistant to oxacillin are resistant to all beta-lactams, with few exceptions (e.g., ceftaroline). Many staph produce a biofilm that helps protect them.
Extended-Spectrum Beta-Lactamase (ESBL)–Producing Organisms: Many gram-negative species of bacteria have been found to possess acquired resistance to beta-lactam antibiotics through the production of beta-lactamase. This enzyme deactivates the agent’s antimicrobial properties by hydrolyzing its beta-lactam ring. The first beta-lactamase to be isolated was a penicillinase identified in E coli in 1940, before penicillin entered medical use. New agents were developed over the years to resist the effects of this enzyme, including broad-spectrum cephalosporins. Predictably, the introduction of these antibiotics led to resistance against broader-spectrum agents, giving rise to the ESBL enzyme. Currently, more than 150 ESBLs are found in many different Enterobacteriaceae species and in P aeruginosa.
Carbapenems have remained the drug of choice for ESBL-producing pathogens. This beta-lactam antibiotic subgroup includes meropenem, ertapenem, doripenem, and imipenem-cilastatin. All of these agents—with the exception of ertapenem, which does not have activity against Acinetobacter or Pseudomonas—have demonstrated efficacy against ESBLs. Similar to the selective development of ESBL enzymes following the introduction of broad-spectrum cephalosporins, there has been an emergence of carbapenem-resistant pathogens capable of hydrolyzing carbapenems through the activity of a carbapenemase enzyme.
Carbapenemase Enzyme: Carbapenems are the broadest-spectrum antibiotics available and are favorable choices for many resistant gram-negative nosocomial infections. As effective as these agents are, there has been an increase in carbapenem-resistant organisms as a result of carbapenem overuse, leaving patients with limited to no treatment options. Carbapenem-resistant Enterobacteriaceae (CRE) and Acinetobacter are associated with a combined hospitalization of 21,600 patients and 1,800 deaths each year in the U.S.6 Other possible carbapenemase-producing organisms include K pneumoniae, P aeruginosa, and E coli.
In order to preserve these antibiotics, carbapenem-sparing agents with activity against ESBL-producing pathogens should be used whenever possible. Such agents include piperacillin-tazobactam, cefepime, and ceftolozane-tazobactam. However, carbapenems are preferred for certain infection sites, including the bloodstream and lungs. In the MERINO trial, 30-day mortality was higher in patients with ESBL E coli or K pneumoniae–associated bacteremia that was treated with piperacillin-tazobactam versus a carbapenem.
Much effort has gone into developing new antibiotics to combat these bacteria. A new type of cephalosporin, cefiderocol, which was approved in 2019 to treat UTIs, has activity against CRE. Recent beta-lactam combinations (meropenem-vaborbactam and ceftazidime-avibactam) have shown good in vitro activity against CRE and are becoming available on many hospital formularies to treat UTIs, intrabdominal infections, and respiratory tract infections. The polymyxins (colistin and polymyxin B), an older antibiotic class, have fallen out of favor because of their renal and neurologic toxicities; however, they are effective against carbapenem-resistant pathogens and may be useful for salvage therapy. Both polymyxin agents are highly similar and have a narrow therapeutic window, requiring careful dosing and close monitoring of renal function, although polymyxin B is associated with less nephrotoxicity than colistin.
Pseudomonas aeruginosa: Pseudomonas is an opportunistic gram-negative pathogen responsible for a large number of nosocomial infections. Antimicrobial agents used to treat Pseudomonas include beta-lactams, fluoroquinolones, and aminoglycosides, but this genus can display multiple mechanisms of resistance. Intrinsically, Pseudomonas has a relatively low membrane permeability (up to 100 times lower than that of E coli). The acquired-resistance mechanisms potentially expressed by Pseudomonas include any of the described strategies. Fluoroquinolones exhibit antimicrobial activity by targeting bacterial enzymes involved in DNA replication.Fluoroquinolone-resistant strains of Pseudomonas avoid this effect by mutating the genes encoding for those enzymes. Aminoglycoside-resistant Pseudomonas is capable of producing aminoglycoside-modifying enzymes. Beta-lactam resistance in Pseudomonas is due to modifications in penicillin-binding proteins, overexpression of efflux pumps, and enzymatic drug inactivation. Pseudomonas has multiple intrinsic β-lactamases including Metallo-B-lactamase and NDM and inducible cephalosporinases, OXA-10 (extended spectrum cephalosporins). It can display carbapenem resistance through modifications of the OprD porin necessary for entry of carbapenem into bacteria. The MexXY efflux pump moves aminoglycosides, fluoroquinolones, tetracyclines, cefepime, tigecycline across the membrane, while the MexAB can move carbapenems (mostly meropenem), antipseudomonal β-lactams, tetracyclines, cefepime and tigecycline out. Pseudomonas also produces a biofilm and has quorum sensing.
Increased exposure of Pseudomonas to an antipseudomonal beta-lactam antibiotic has been shown to increase the risk of developing resistance. In a retrospective cohort study of 7,118 patients, each additional day of antipseudomonal beta-lactam therapy was associated with a 4% increased risk of new resistance at 60-day follow-up. To avoid continuing resistance, diligent efforts should be made to limit beta-lactam exposure to the shortest effective duration.
Enterobacteriaceae/Enterobacteriales: When dealing with Enterobacteriales it is important to differentiate 2 major groups. E. coli, K. pneumoniae, K. oxytoca & P. mirabilis are the group that lack intrinsic resistance to extended spectrum cephalosporins. Their wild-type beta-lactam pattern is considered pan-susceptible except for K. pneumoniae and K. oxytoca that are resistant to ampicillin by the presence of plasmid-mediated TEM/SHV.
The second group, commonly referred to as SPiCE/SPACE (or now, HECK-YES) includes Serratia marcescens, Providencia stuartii, Citrobacter spp., Enterobacter spp., and Morganella morganii but now Hafnia alvei, Enterobacter cloacae, Citrobacter freundii, Klebsiella aerogenes, and Yersinia enterocolitica are the more common species (see Kohlmann R, Bähr T, Gatermann SG. Species-specific mutation rates for ampC derepression in Enterobacterales with chromosomally encoded inducible AmpC β-lactamase. J Antimicrob Chemother. 2018 Jun 1;73(6):1530-1536. doi: 10.1093/jac/dky084. PMID: 2956614). These organisms (except for some species in the Enterobacter and Citrobacter genera) carry a chromosomal cephalosporinase commonly referred as AmpC. This group of genes belongs to the Ambler Class C and they are not inhibited by clavulanic acid, sulbactam or tazobactam (with some exceptions). The spectrum of activity for AmpC does not include cefepime. Many penicillins and first- to third-generation cephalosporins do not show resistance, but these agents activate ampC and will induce resistance.
The presence of ampC is not detectable by most laboratories, but if resistance to cefoxitin is displayed on culture and sensitivity reports, this can serve as a surrogate marker for ampC, in which case penicillins and narrow-spectrum cephalosporins should be avoided.
Aside from AmpC, Enterobacterales may have porin and efflux pump modifications may result in increases in MICs, though may not lead to frank resistance alone. They may have fluoroquinolone resistance due to target site alterations or efflux pumps, but this can also be plasmid mediated. They may have tetracycline resistance often due to efflux pumps or target site alterations (ribosomal protection). Polymyxin resistance most often due to alterations in lipid membrane. Many enterobacterales produce a biofilm (including E. coli, Klebsiella pneumoniae, Proteus mirabilis).
Streptococci:: Ribosome methylation causes azithromycin resistance. Alterations in DNA gyrase and topoisomerase II cause fluoroquinolone resistance. β-lactam resistance most often due to PBP alterations. Many streptococci produce a biofilm.
A. baumanni: Intrinsic production of β-lactamases including Metallo-β-Lactamase, OXA-51 (can cause carbapenem resistance), OXA-23/24 (carbapenem resistance), AmpC. Multiple porins and efflux pumps associated with resistance have been identified. Sulbactam resistance thought to be a result of PBP alterations
Stenotrophomonas maltophilia: S. maltophilia has Intrinsic production of a metallo-β-lactamase and a cephalosporinase (L1 and L2, respectively). It can be multidrug resistance via efflux pumps Trimethoprim resistance generally results from production of dihydrofolate reductase. Sulfamethoxazole resistance is usually due to dihydropteroate synthase alterations
Some “Fun” Virulence Factors
Bactiria have a few mechanisms of evading host defenses. These may also make treating them harder. They typically fiit into 3 groups:
- Adherence Factors: Many pathogenic bacteria colonize mucosal sites by using pili (fimbriae) to adhere to cells. Neisseria gonorrhoeae, Neisseria meningitidis, Escherichia coli, and Pseudomonas aeruginosa all have pili.
- Invasion Factors: These surface components that allow the bacterium to invade host cells can be encoded on plasmids, but more often are on the chromosome.
- Endotoxins: The lipopolysaccharide endotoxins on Gram-negative bacteria cause fever, changes in blood pressure, inflammation, lethal shock, and many other toxic events. E. coli, Salmonella, Shigella, Vibrio cholerae, and Neisseria meningitidis are some bacteria that produce endotoxins.
- Capsules: Many bacteria are surrounded by capsules that protect them from opsonization and phagocytosis. Acapsule is found most commonly among gram-negative bacteria: Escherichia coli (in some strains) Neisseria meningitidis. Klebsiella pneumoniae.
- Exotoxins: Exotoxins include several types of protein toxins and enzymes produced and/or secreted from pathogenic bacteria. Major categories include cytotoxins, neurotoxins, and enterotoxins.The various forms of Clostridia are prime examples of bacteria that produce exotoxins. Some Gram-negative bacteria, such as Pseudomonas aeruginosa, produce exotoxins as do most Gram-positive bacteria. Toxic shock syndrome is caused by staphylococcal or streptococcal exotoxins.
- Siderophores: Siderophores are iron-binding factors that allow some bacteria to compete with the host for iron, which is bound to hemoglobin, transferrin, and lactoferrin. They are produced in response to iron deficiency by Gram-positive and Gram-negative bacteria.
The Bacterial Community
Bacteria also have some factors that enhance bacterial community fitness.
Quorum Sensing
Quorum sensing is a mechanism employed by bacteria to communicate and coordinate their behavior based on population density. This process involves the production and detection of signaling molecules, allowing bacteria to regulate gene expression collectively. For example, some microbes won’t produce toxins until their population is high enough to evade host response. It’s like waiting for a fight until your buddies show up as backup.
Quorum Sensing Mechanisms: Bacteria use quorum sensing to regulate a wide array of physiological activities, including biofilm formation, virulence factor expression, and antibiotic resistance. Through this mechanism, bacteria can synchronize their activities to enhance their survival and pathogenicity. The coordinated action of bacteria in response to quorum-sensing signals can lead to the formation of biofilms, which are notorious for their resistance to antimicrobial agents.
Impact on Antimicrobial Resistance: Quorum sensing has been found to play a role in the development of antimicrobial resistance by facilitating the exchange of genetic material, upregulating efflux pumps, and modulating the expression of resistance genes. Additionally, the activation of quorum sensing pathways can lead to the development of tolerance to antibiotics, enabling bacterial populations to survive and thrive in the presence of antimicrobial agents.
Biofilms
Biofilms are complex structures formed by microorganisms, which can have significant implications for antimicrobial resistance and human health. These biofilms are composed of bacterial colonies or single types of cells that adhere to surfaces and are embedded in an extracellular polymeric matrix. This matrix, consisting of substances like eDNA, proteins, and polysaccharides, provides high resistance to antibiotics, making biofilm-associated infections challenging to treat.
Biofilm Formation and Resistance: Biofilms are known to increase the resistance of microorganisms to antimicrobial agents. Bacteria living in biofilms can exhibit a 10 to 1,000-fold increase in antibiotic resistance compared to similar bacteria living in a planktonic state. The extracellular polymeric substance (EPS) layer of biofilms can trap antibacterial drugs, reducing their effectiveness against the organisms present in the biofilm.
Synergy
Synergy is often defined as when the effect of two or more agents is greater than the sum of the individual agents and often used to overcome resistance. Typically, it is suggested to give the Beta-lactam first and then give the other antibiotic fairly soon after. This has been shown to decrease mortality. Beta-lactams can bind to the PBP enzymes and allow the other antibiotic to penetrate better, plus are normally more effective than the second drug.
Many combinations have in vitro synergy, with complementary mechanisms of action:
- Carbapenems and polymycins
- Polymyxin monotherapy often discouraged due to regrowth of bacteria within 24 hours in in vitro studies
- β-lactams + aminoglycosides
- Aminoglycosides generally not used as monotherapy in severe infections, like endocarditis
- β-lactams + daptomycin
- May be used for persistent staphylococcal infections or those with reduced susceptibility to daptomycin
- Exploits “seesaw” effect
- As daptomycin MIC increases, β-lactam MICs decrease due to cell wall thickening and increased usage of PBPs
- “Seesaw” effect also observed with vancomycin and β-lactams
- Dual β-lactam synergy has been demonstrated in some cases
- Ampicillin + ceftriaxone
- Ceftazidime-avibactam + aztreonam
- Potentially active against metallo-β-lactamase producers
- Aztreonam stable against MBLs, avibactam provides protection against co-expressed βlactamases (e.g., ESBLs, KPC, AmpC)
- Ertapenem and meropenem or doripenem
- Ertapenem acts as a “suicide substrate,” is hydrolyzed by carbapenemases
- Second carbapenem is allowed to access active site
- In this case, ertapenem should be administered first
- Ceftriaxone + ampicillin
- Increasingly used for ampicillin-susceptible enterococcal endocarditis
- Use of these agents overwhelms PBPs, leading to bactericidal activity
- Similar clinical outcomes to ampicillin + gentamicin with significantly lower risk of adverse events
There is some evidence that the Beta-lactam needs to come before other drugs when used in combination (or for synergy). There could be many reasons for this: modifying the cell wall could allow for easier entry of the other drug for example, but it’s likely that beta-lactams are just usually more effective and more likely to be susceptible, so hitting with the better drug first allows quicker recovery. See Joe Amoah et al., “Administration of a β-Lactam Prior to Vancomycin as the First Dose of Antibiotic Therapy Improves Survival in Patients with Bloodstream Infections,” Clinical Infectious Diseases, no. ciab865 (October 4, 2021).